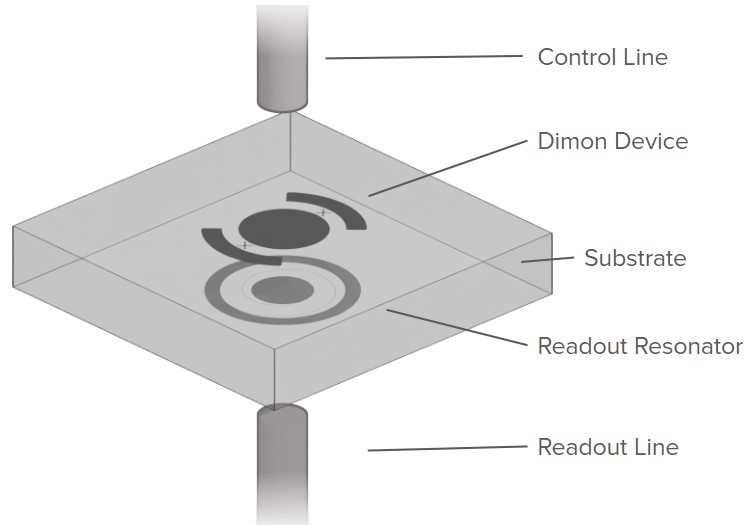
TECHNICAL BLOG
Hardware efficient error-detection
- Error detection and correction needs to be introduced to improve computations. Due to the complex behaviour of physical quantum computers, these error reduction schemes can take many forms.
- OQC have recently demonstrated a Hardware efficient error-detection method for reducing the most significant source of error in our quantum computers.
- We built a variant of the Coaxmon based on the ‘Dimon’. We encode a logical qubit in the single-photon excitation subspace of the dimon, using the method of dual-rail encoding.
- The OQC Dimon introduces a novel approach without the need for additional hardware or wiring and preserves the compact footprint and control wiring density of the Coaxmon, while enhancing error detection capabilities.
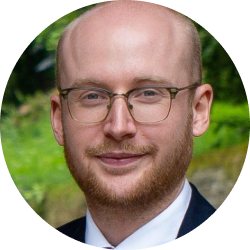
James Wills
QUANTUM ENGINEER
James completed his PhD in Physics at the University of Oxford, in the group of Peter Leek. He worked on the design, simulation, and measurement of novel coaxial qubits, specialising in multi-mode coaxial transmons for quantum computing and sensing applications. He joins OQC as a Quantum Engineer, working in the Quantum Research and Development team.
Despite incredible progress from quantum engineers around the globe, a fundamental challenge remains to produce scalable, low-error quantum computers. Error detection and correction needs to be introduced to improve computations. However due to the complex behaviour of physical quantum computers, these error reduction schemes can take many forms. Here at OQC we have recently demonstrated a hardware efficient method for reducing the most significant source of error in our quantum computers. We believe this is an essential path forward to achieve the reliability and scalability necessary for practical quantum computing applications.
Why do we need error correction?
Error correction in the context of computing, including quantum computing, refers to the process of identifying and rectifying errors that occur during data storage or processing. In quantum computing, error correction is even more crucial due to the nature of quantum states and the susceptibility of qubits to disturbances from their surrounding environment. Unlike classical bits, where errors typically involve bit flipping, a 1 to 0 or a 0 to 1, quantum computing has to grapple with many other errors such as phase flips, exacerbated by noise and decoherence.
Superconducting qubits are a promising architecture for quantum computers due to their straightforward fabrication and flexible design; however like all practical quantum computers, they are still prone to errors in a computation. One such error mechanism is energy relaxation (or T1 decay), where a qubit in its excited state (1) will decay to the ground state (0). This can be a result of a variety of reasons such as material defects, coupling to lossy circuit elements, or even ionising or cosmic radiation. It is a common goal of many researchers, both academic and industrial, across the world to reduce this error mechanism.
Correcting these errors demands encoding schemes capable of identifying error types and locations swiftly – a challenge yet to be solved.
The cost of implementing error correction
Quantum error correction (QEC) requires redundant encoding. Encoding just one single logical qubit (e.g error-corrected qubit) across a vast array of physical qubits is a foundational step, but at scale the challenges are very real.
These quantum error correcting codes require a large number of physical qubits to protect the quantum information, with thousands of physical qubits potentially required for a single reliable logical qubit. When you reach this quantity of qubits, you begin to face aggressive engineering challenges; from your fridge design, to how you wire your sample holders.
A significant constraint in quantum computing lies in how we scale, as increased scalability reduces the thermal load and mass on dilution refrigerators. For many modalities of quantum computing, using cryogenics is instrumental to being able to achieve the necessary conditions for reducing decoherence and minimising noise.
A dilution refrigerator is a cryogenic platform that can cool bulk materials to temperatures around 10 mK (10 thousandths of a degree above absolute zero).
Eventually, the demand for scalability will surpass the cooling capacity of fridges: our approach not only enables us to address errors, but also ensures the future of integrating quantum computing within existing commercial data centres.
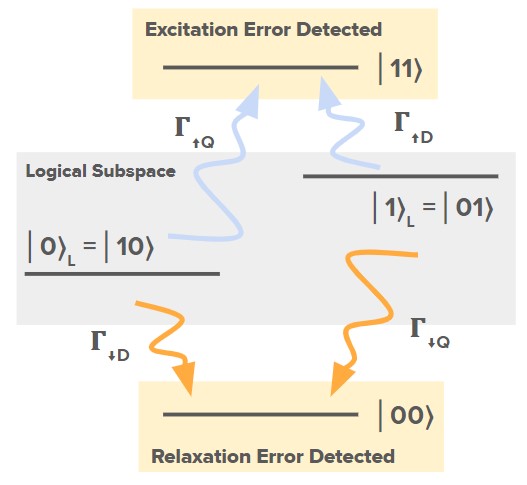
Hardware efficient error-detection: dual-rail encoding in a multimode coaxial transmon
We built a variant of the Coaxmon, based on the ‘Dimon’, and while Dimons aren’t new devices – our approach is. We encode a logical qubit in the single-photon excitation subspace of the dimon, using the method of dual-rail encoding. Any energy relaxation event of the physical qubit modes results in a leakage outside of the computational subspace, which we can efficiently detect as an erasure error. These errors can be flagged and removed in post processing, resulting in an energy relaxation free measurement result.
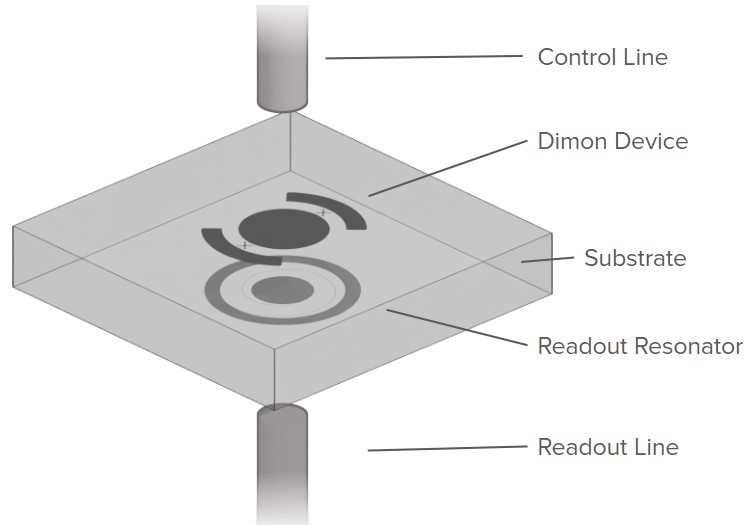
Figure: Control and readout is achieved via coaxial control pins. The device consists of three superconducting islands, two Josephson junctions and has a readout resonator on the opposite side of the substrate.
Erasure error-detection is a new and exciting prospect for superconducting quantum devices. Borrowing from techniques previously explored by neutral atom technologies, the methods can result in a reduction of hardware overhead required for error-correction, or be a path to more complex and efficient encoding methodologies.
The OQC Dimon introduces a novel approach without the need for additional hardware or wiring and preserves the compact footprint and control wiring density of the Coaxmon, while enhancing error detection capabilities. This hardware efficiency makes the device a promising candidate for more complex and optimised error-correction methodologies, without further increasing the physical qubit overhead.
The novelty lies in the hardware efficiency, and smaller physical footprint, making it even more scalable
SNAPSHOT: What is a dimon?
Where a conventional qubit has two energy levels, forming the computational 0 and 1, a dimon is a more complex device. Consisting of three superconducting islands, and two Jospehson junctions, the device has an additional addressable normal mode, and a V-shaped energy level structure.
Over the years, dimons, and multimode qubits in general, have been widely researched for a number of applications. These range from suppressing dephasing and relaxation mechanisms, to novel coupling and entanglement generation methods. It is the versatility of superconducting circuits that allows the engineering of these complex structures for practical purposes.
Initial results are promising
We have shown an improvement in conventional qubit coherence measurements (T1, T2E), demonstrating lower error-rates than the non-error detected components. For short circuit depths, we observe a 60x improvement in bit-flip error rates, and 15x improvement in phase-flip error rates. Beyond this we are observing physical limits not observed in conventional qubits, such as second order decay and re-excitation processes, that can be resolved with further measurement processes.
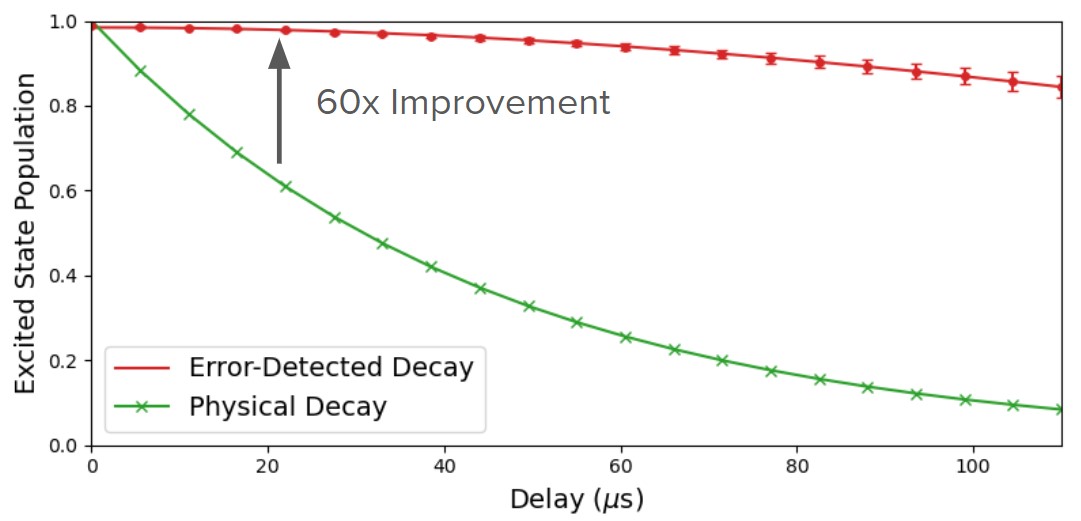
Figure: Bit-flip error measurement of the device without error-detection (green) and with erasure error-detection (red). Error-detected measurement shows a non-exponential decay profile, with significant improvements in error-rate over the non-error detected measurement at short circuit depths.
We show in an animation of a qubit coherence measurement, oscillations occur between the logical states 0L (green) and 1L (orange), with the volume of erasure error-detected measurements (blue) exponentially increasing. In a normal qubit, the signal would decay after 30-40 microseconds. In our device, following the error-detection and post-selection processing, there is no longer an exponential decay, and coherent oscillations persist beyond 100+ microseconds.
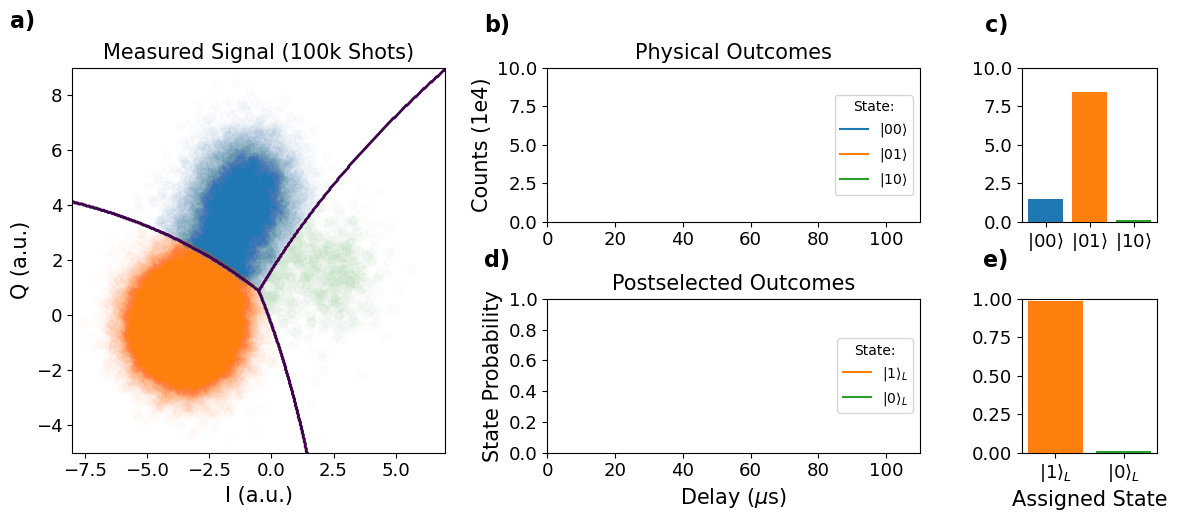
Whilst being a potential building block for more advanced quantum error correction methods, our experiment is allowing us to probe into the fundamental physics of what causes decoherence in protected logical subspaces. Understanding of these mechanisms is vital for the future of superconducting qubits research and will allow us to make improvements in our conventional qubit devices.
Potential future applications and directions
We believe the dimon is a building block for novel quantum error correction, and have demonstrated the first steps towards this with dual-rail encoding and erasure error-detection. Our results show an order of magnitude improvement in conventional coherence metrics, whilst retaining the inherent hardware efficiency and physical footprint of the coaxmon.
Join our newsletter for more articles like this
By clicking ‘sign up’ you’re confirming that you agree with our Terms & Conditions